Give your clients a personalized wellness music experience.
guide
The ABC of Music in Exercise: Affect, Behavior, and Cognition
A White Paper by:
Costas I. Karageorghis
Brunel University London, UK December 2021
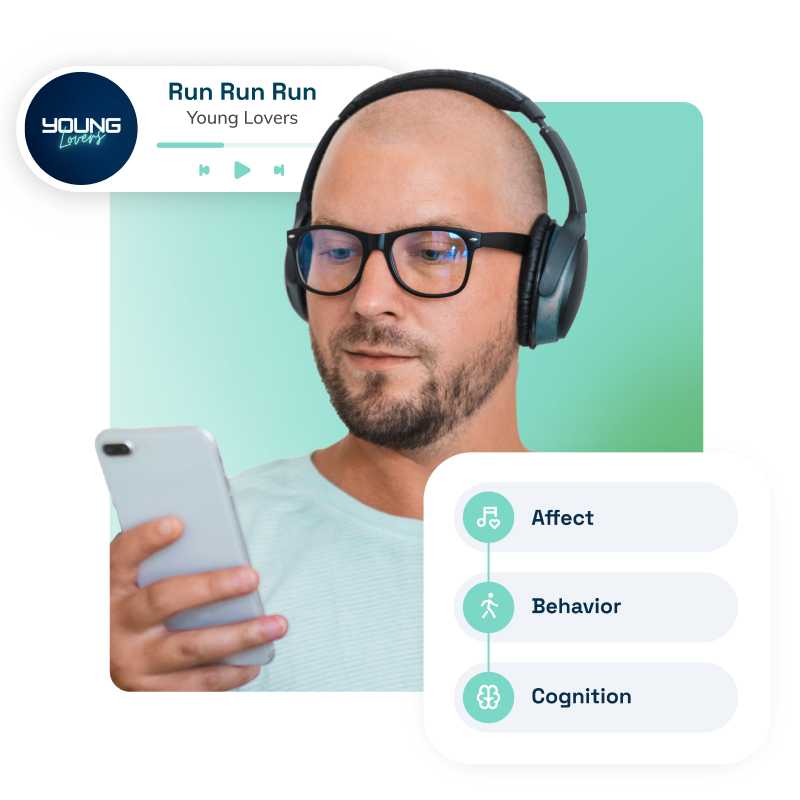
One good thing about music, when it hits you, you feel no pain. - Bob Marley
Introduction
The human race appears to be hardwired to produce, appreciate, and move in time to music (Levitin, 2007). Anthropologists suggest that music forms part of our genetic blueprint (Mithen, 2005). Through the eons, music has been integrated into many facets of our private and communal lives. In recent decades, one cannot fail to notice just how pervasive music-related interventions have come to be in the realm of health and fitness. With the cataclysm that has been the COVID–19 global pandemic and attendant restrictions on social activity, the bond between music and exercise has been considerably strengthened (Kaur et al., 2020).
In this white paper, I expound the purpose and application of music in the exercise and physical activity domain. The golden thread is the influence of music on affect (underlying emotion), behavior (exercise performance), and cognition (thought processes); hence the titular “ABC”.
The paper begins with an outline of key concepts, reviews recent research that has examined the ABC, details the neurophysiological mechanisms that underlie the effects of music in exercise, and concludes with evidence-based guidance on application.
Professor Costas I. Karageorghis PhD,
CPsychol, CSci, FBASES, AFBPsS
Professor of Sport & Exercise Psychology
Divisional Lead for Sport, Health & Exercise Sciences Department of Life Sciences
College of Health, Medicine & Life Sciences
Brunel University London, UK
@SAVIBrunel
The nature of music
Any piece of music is characterized by the combination of four key elements: melody, harmony, rhythm, and dynamics. Melody is the highest-pitched part, to which one might whistle along. Harmony involves the simultaneous sounding of tones that serves to project the ‘mood’ of the music (e.g. happy or sad). Rhythm pertains to the speed or rate of music and how its various components are accented as it unfolds over time. This element can prompt an immediate physical reaction from the listener. Dynamics concern the energy transmitted by musicians through their instruments—an energy modulated by breath or touch. A basic understanding of these elements facilitates exercise practitioners and technologists in harnessing the power of music (Karageorghis, 2017).
How music can be applied to exercise
Music can be applied to exercise in three primary ways: pre-task, in-task, and post-task (Terry & Karageorghis, 2011). The in-task application can be further broken down into synchronous and asynchronous uses (Lim et al., 2014), while the post-task application can be split into respite and recuperation uses (Jones et al., 2017; Karageorghis et al., 2018a). The synchronous use entails the exerciser moving in strict time with music, while in the asynchronous mode, this does not happen (Karageorghis & Terry, 2009). Respite music applications pertain to exercise modalities such as High-Intensity Interval Training (HIIT), wherein music is used to enhance exercise-related affect during recovery periods (Karageorghis et al., 2021a). Recuperative music is used at the very end of an exercise session to facilitate recovery and revitalization (Jing & Xudong, 2008; Karageorghis et al., 2018a).
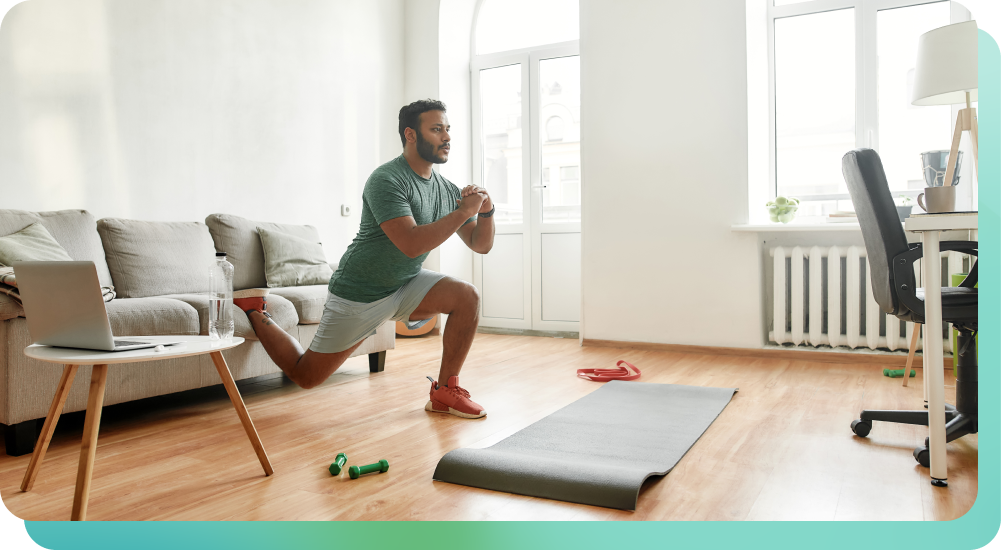
The ABC of musical responsivity in exercise
Pre-task music serves a ‘psych-up’ or ‘psych-down’ function, which means that it can alter an exerciser’s affective or emotional state. It can thus be used to mentally prepare an exerciser or induce an optimal level of activation for what is to come (Karageorghis et al., 2018b; Smirmaul, 2017). Pre-task music can also elicit exercise-related cognitions or thought processes that feed into mental preparation; among such cognitions might be exercise-related imagery or positive self-talk prompted by lyrical excerpts (e.g. “I feel good”, “Let’s get physical”, or “Moving on up”).
Music can temper the shift toward negative affect (i.e. displeasure) that is unavoidable during intense forms of exercise
People seldom need relaxing music prior to an exercise session (unless they are anxious about going to the gym); rather they need tracks that will engender an active state (see Karageorghis & Priest, 2012). With in-task usage, a key role of music is to elevate affect and lower the exerciser’s perception of exertion. Music can temper the shift toward negative affect (i.e. displeasure) that is unavoidable during intense forms of exercise (Bigliassi, Karageorghis, Nowicky, Orgs & Wright, 2016; Jones et al., 2017; Karageorghis et al., 2021a). Typically, during the asynchronous application of music, the rating of perceived exertion (RPE) is lowered by 10% at low-to-moderate intensities of exercise.
Staying with in-task music but focusing specifically on its use in the synchronous mode, there can be behavioral benefits in addition to affective and cognitive benefits. Foremost among these is that the synchronous application of music can have an ergogenic or work-enhancing effect; this has been demonstrated in track running (Karageorghis et al., 2019; Simpson & Karageorghis, 2006), treadmill running (Bood et al., 2013; Terry et al., 2012), and circuit-type exercise (Karageorghis et al., 2010). Among people who are recreationally active, the ergogenic effect can be as great as 15% (Karageorghis et al., 2009). Notably, people who take their fitness training very seriously may have well-established work rates, and are thus less inclined to alter their movements to keep time with a musical beat.
Research into post-task music is at a relatively early stage but it has been shown that medium-tempo (120–125 bpm) respite–active music (i.e. music used for active recovery) applied to a HIIT protocol improved affective valence (i.e. how good people felt) during exercise bouts and active recovery (Karageorghis et al., 2021a). Both medium- and fast-tempo (135–140 bpm) music increased dissociation, exercise enjoyment, and remembered pleasure when compared to a no-music control. In addition, medium-tempo music was shown to reduce RPE during recovery periods, which represents a cognitive benefit.
In recent work that examined recuperative music (i.e. music applied after exercise), it was shown that slow, sedative music (ave. tempo = 71 bpm) facilitated reductions in the arousal dimension of affect when compared against no-music control and fast, stimulative music (ave. tempo = 129 bpm) conditions (Karageorghis et al., 2018a; see Figure 1). Interestingly, women had a more pronounced reduction in affective arousal than men in response to slow, sedative music. Also, the same music prompted more positive affective valence responses when compared with the no-music control and fast, stimulative music conditions.
Figure 1. A significant Condition × Time interaction (p < .001) showing that slow, sedative music facilitates reductions in affective arousal during active and passive recovery from exhaustive cycle ergometer exercise (Karageorghis, Bruce, Pottratz, Stevens, Bigliassi, & Hamer, 2014). Adapted from Medicine & Science in Sports & Exercise 50(4), C. I. Karageorghis, A. C. Bruce, S. T. Pottratz, R. C. Stevens, M. Bigliassi, & M. Hamer, “Psychological and psychophysiological effects of recuperative music postexercise,” 739–746, 2018, with permission from Lippincot Williams & Wilkins. https://journals.lww. com/acsm-msse/Fulltext/2018/04000/Psychological_and_Psychophysiological_Effects_of.13.aspx
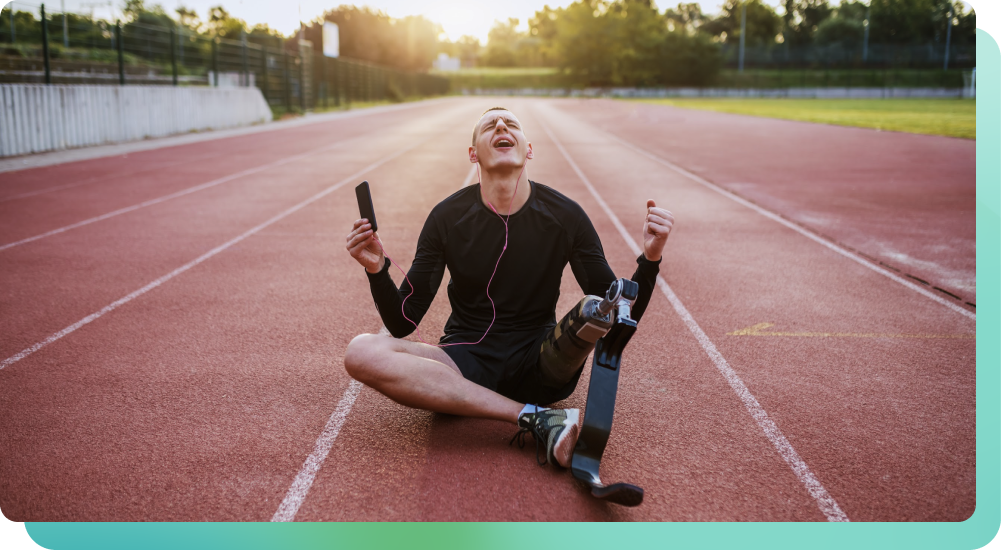
Neurophysiological mechanisms underlying the effects of music in exercise
Neurophysiology is a branch of physiology and neuroscience that focuses on the functioning of the nervous system. The neurophysiological mechanisms that account for the ways in which music influences exercise participants have only begun to attract the attention of researchers over the last decade. Technologies that have evolved rapidly, such as functional magnetic resonance imaging (fMRI), functional near-infrared spectroscopy (fNIRS), and mobile electroencephalography (EEG) units, have greatly facilitated scientists’ exploration of such mechanisms (Bigliassi et al., 2018, 2019; Karageorghis et al., 2017, 2018c).
Attentional dissociation
When exposed to music, an exerciser will experience a shift in attention from internal, bodily-related cues to the pleasurable external cue that music provides (Clark et al., 2016; Hutchinson et al., 2015). The shift can be explained, in part, by the limited capacity of the afferent nervous system, which is responsible for the inward transmission of sensory impulses toward the brain and spinal column (i.e. the central nervous system). The shift is linked with the ~10% reduction in perceived exertion during exercise at a low-to-moderate intensity (Clark et al., 2016; Karageorghis, 2017). At high exercise intensities (i.e. beyond 75% of aerobic capacity; VO2max), the afferent signals from the musculature and vital organs become overwhelming in attentional terms, and so music is far less effective in reducing perceived exertion (Terry et al., 2020).
Figure 2 illustrates how fast-tempo pop music can influence associative (task-related) and dissociative (task-unrelated) thoughts at six exercise intensities (40–90% of maximal heart rate reserve; maxHRR). In this study (Karageorghis & Jones, 2014), the music decreased associative thoughts and increased dissociative thoughts at low, moderate, and high exercise intensities. The attentional shift (see arrow in Figure 2) means that the crossover of dissociation to association is displaced to the right and occurs at ~80% maxHRR with music, rather than at ~70% maxHRR without music. The main implication of this shift is that people can be distracted by music at exercise intensities at which they are likely to accrue significant cardiometabolic benefits (e.g. reduced risk of coronary heart disease).
Figure 2. Comparison of state attention scores across all exercise intensities between no-music control and fast-tempo music conditions (Karageorghis & Jones, 2014). Adapted with permission from C. I. Karageorghis & L. Jones, “On the stability and relevance of the exercise heart rate music-tempo preference relationship,” 2014, Psychology of Sport and Exercise, 15, 307. Reproduced with permission of Elsevier.
https://www.sciencedirect.com/science/article/pii/S1469029213000836
Touching the pleasure center of the brain
Music is an aesthetic stimulus that promotes pleasure and, by extension, can enhance the affective or emotional experience of exercise. Neuroimaging studies that use fMRI have shown that the nucleus accumbens—part of the brain’s reward circuit (see Figure 3)—pertains to a mechanism that underlies the influence of music on affective state (Zatorre & Salimpoor, 2013). Music might improve the exercise-related affect through elevating dopamine levels in the nucleus accumbens. Dopamine is a neurotransmitter that has multiple functions, one of which relates to regulating the response of the brain to rewarding or pleasant stimuli.
Music might improve the exercise-related affect through elevating dopamine levels in the nucleus accumbens
There are multiple studies showing that, even during high-intensity exercise, music can elicit more positive affect (Jones et al., 2014; Karageorghis et al., 2009). It is thought that the mechanisms that underlie such brain responses are related to the activity of subcortical structures, such as the amygdala and anterior cingulate cortex (Koelsch, 2014; see Figure 3). At high exercise intensities, music can have a meaningful effect with little higher-cortex processing taking place. This is because pleasurable stimuli direct the activity across a range of sensory pathways in tandem (Blood & Zatorre, 2001; Leknes & Tracey, 2008). Moreover, EEG studies show that exercise consciousness is reduced and there is less electrical connectivity among areas of the brain responsible for communicating fatigue when music is present (Bigliassi et al., 2016, 2017).
Figure 3. An illustration of the nucleus accumbens, amygdala, anterior cingulate cortex, premotor, and cerebellar regions of the human brain.
The ‘rhythm response’
There is a well-established ‘rhythm response’ associated with the use of music during exercise (e.g. Ramji et al., 2016). This response relates to an innate predisposition humans have to synchronize movement with the rhythmical qualities of music (Levitin, 2007). A neurophysiological explanation for this resides in similarities between movement rate during exercise and preferred music tempo that is reflected by the frequency of EEG delta wave or slow wave activity (~3 Hz; Schneider et al., 2010). It has been suggested that there is a “central pattern generator” in the brain that provides a neural platform for rhythmicity in movement (MacDougall & Moore, 2005, p. 1172).
Research using fMRI has illustrated the engagement of pre-motor and cerebellar brain regions (see Figure 3) when preferred musical rhythms are heard, as compared to non-preferred ones (Kornysheva et al., 2010). It is plausible that such brain activity facilitates the process of “locking in” to a musical beat that an exerciser finds appealing. It is notable that the use of music in the synchronous mode can reduce the metabolic cost of exercise, as it promotes greater neuromechanical efficiency (Bacon et al., 2012; Maes et al., 2016).
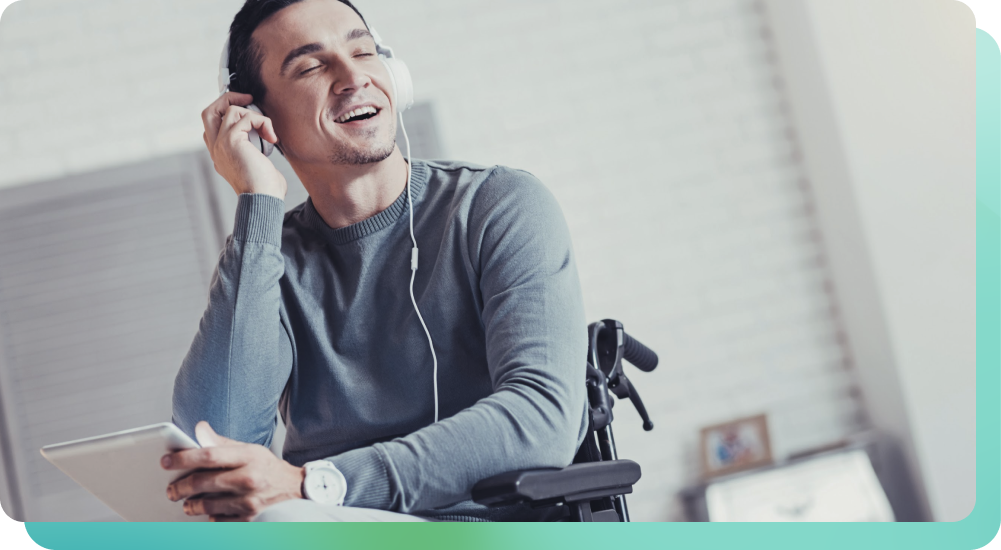
Recommendations for practice
A primary consideration is that music selected to accompany exercise should
be congruent with exercisers’ personal preferences—incorporating age-related factors and socio-cultural background—and meet the demands of the intended exercise task. Algorithm-based streaming that draws upon the preferred tracks of an individual, as well as the ‘near neighbor’ tracks in stylistic terms, provides a good point of origin in this regard. The music program should also be targeted at the desired affective, behavioral, and cognitive consequences (i.e. the ABC) for a given type of workout.
A primary consideration is that music selected to accompany exercise should be congruent with exercisers’ personal preferences and meet the demands of the intended exercise task.
Targeting the ABC might relate to arousing the exerciser before a session, distracting them or providing a metronomic-type function during exercise, and returning the exerciser to a calm and revitalized state after their workout. The program could also provide an end-to-end solution that covers all elements of a given exercise program and so serves to ‘contour’ the physiological peaks and troughs (Hallett & Lamont, 2017; Priest & Karageorghis, 2008). Moreover, full advantage should be taken of lyrical content to provide affirmation, empowerment, and motivation (Sanchez et al., 2014).
Music tempo should be administered with the desired exercise intensity in mind. Other than for warmup and warm-down phases, or recovery/recuperation, the tempo “sweet spot” in the asynchronous application is ~120–140 bpm (Karageorghis & Jones, 2014; Karageorghis et al., 2011). It is also advantageous for the rhythm of the music to approximate the motor patterns that typify a given type of exercise activity (Karageorghis, 2017; Leman et al., 2013). For example, a waltz-type rhythm characterized by three beats to the bar with the first beat accented (i.e. a 3/4 time signature), is ideal for the front crawl in swimming. This is because there is a strong kick to every arm stroke that is followed by two softer kicks—a one two three, one two three rhythmic feel.
In the assignation of pre-task music, practitioners and technologists can take advantage of extra-musical associations. For example, music that reminds people of an action hero (e.g. Rocky Balboa), a popular TV sports show (e.g. the famous BBC Wimbledon theme), or an exercise-related music-video (e.g. Eric Prydz’s Call On Me). With reference to in-task applications, in recent years, exercise machine–digital music interfaces have been developed that automatically shift the tempo of music or change a musical selection to adapt in precise terms to an exerciser’s movement rate (see e.g. Moens et al., 2014; D-Jogger).
The pandemic has severely impacted people’s patterns of physical activity in the Western world and digital music-based solutions provide a means by which to make exercise a habitual activity and for it to be rendered more enjoyable.
This approach is also possible in free-form exercise that uses adaptive technology, which is designed to augment and intensify a music-listening experience. Specifically, pieces of music with several tracks or layers can change in tempo, energy, and orchestration to match movement patterns that are assessed by a smartphone accelerometer. This is very different to conventional music streaming services that deliver non-adaptive music (i.e. it always sounds the same). Such digital advancements afford exercisers a wraparound music solution to optimize the ABC outcomes.
The pandemic has severely impacted people’s patterns of physical activity in the Western world (Karageorghis et al., 2021b; Wilke et al., 2021) and digital music-based solutions provide a means by which to make exercise a habitual activity and for it to be rendered more enjoyable (Kaur et al., 2020; Shin, 2021). Such solutions can be applied to circuit training or running in the park just as easily as they can be applied to calisthenics or dance aerobics in the home.
Keeping exercise participants’ safety in mind, there are certain contraindications that have been proposed in relation to the application of music in exercise (Karageorghis et al., 2012): (a) when it might distract from safety-relevant information (e.g. while cycling on the roads); (b) when exercisers need to focus their full attention on learning a motor skill (e.g. a complex type of lift with weights); and (c) when exercising at high intensities that require an associative attentional style (i.e. “listening to the body”) to minimize the risk of injury. Moreover, repeated exposure to high-intensity music (> 85 dBA) coupled with high-intensity exercise is to be avoided, given the potential damage that can be caused to the structures of the inner ear (Nakashima et al., 2003).
Appropriate music selections can result in enhanced affect that is accompanied by greater work output and a reduction in perceived exertion
Across a range of exercise contexts and in associated scientific studies, participants appear to experience the ABC consequences of music use in unison. Appropriate music selections can result in enhanced affect that is accompanied by greater work output and a reduction in perceived exertion (Bigliassi et al., 2016; Bood et al., 2013; Karageorghis et al., 2009, 2010; Stork et al., 2015, 2019). It is particularly notable that music is a stimulus that can increase work output while simultaneously reducing perceived exertion (Terry et al., 2020). Therein lies one of its most potent benefits in the realm of exercise. As the global community strives to recover from the pandemic and re-establish positive physical activity habits, it is clear that the exercise–digital music nexus holds considerable value.
Sources:
Bacon, C. J., Myers, T. R., & Karageorghis, C. I. (2012). Effect of music-movement synchrony on exercise oxygen consumption. The Journal of Sports Medicine and Physical Fitness, 52, 359–365.
Bigliassi, M., Karageorghis, C. I., Bishop, D. T., Nowicky, A. V., & Wright, M. J. (2018). Cerebral effects of music during isometric exercise: An fMRI study. International Journal of Psychophysiology, 133, 131–139.
Bigliassi, M., Karageorghis, C. I., Hoy, G. K., & Lane, G. S. (2019). The Way You Make Me Feel: Psychological and cerebral responses to music during real-life physical activity. Psychology of Sport and Exercise, 41, 211–217.
Bigliassi, M., Karageorghis, C. I., Nowicky, A. V., Orgs, G., & Wright, M. J. (2016). Cerebral mechanisms underlying the effects of music during a fatiguing isometric ankle‐dorsiflexion task. Psychophysiology, 53, 1472–1483.
Bigliassi, M., Karageorghis, C. I., Wright, M. J., Orgs, G., & Nowicky, A. V. (2017). Effects of auditory stimuli on electrical activity in the brain during cycle ergometry. Physiology & Behavior, 177, 135–147.
Blood, A. J., & Zatorre, R. J. (2001). Intensely pleasurable responses to music correlate with activity in brain regions implicated in reward and emotion. Proceedings of the National Academy of Sciences, 98, 11818–11823.
Bood, R. J., Nijssen, M., Van Der Kamp, J., & Roerdink, M. (2013). The power of auditory-motor synchronization in sports: Enhancing running performance by coupling cadence with the right beats. PLoS One, 8, e70758.
Clark, I. N., Baker, F. A., & Taylor, N. F. (2016). The modulating effects of music listening on health-related exercise and physical activity in adults: A systematic review and narrative synthesis. Nordic Journal of Music Therapy, 25, 76–104.
Hallett, R., & Lamont, A. (2017). Music use in exercise: A questionnaire study. Media Psychology, 20, 658–684.
Hutchinson, J. C., Karageorghis, C. I., & Jones, L. (2015). See hear: Psychological effects of music and music-video during treadmill running. Annals of Behavioral Medicine, 49, 199–211.
Jing, L., & Xudong, W. (2008). Evaluation on the effects of relaxing music on the recovery from aerobic exercise-induced fatigue. The Journal of Sports Medicine and Physical Fitness, 48, 102–106.
Jones, L., Karageorghis, C. I., & Ekkekakis, P. (2014). Can high-intensity exercise be more pleasant? Attentional dissociation using music and video. Journal of Sport & Exercise Psychology, 36, 528–541.
Jones, L., Tiller, N. B., & Karageorghis, C. I. (2017). Psychophysiological effects of music on acute recovery from high-intensity interval training. Physiology & Behavior, 170, 106–114.
Karageorghis, C. I. (2017). Applying music in exercise and sport. Champaign, IL: Human Kinetics.
Karageorghis, C. I., Bigliassi, M., Guérin, S. M. R., & Delevoye-Turrell, Y. (2018c). Brain mechanisms that underlie music interventions in the exercise domain. In S. Marcora & M. Sarkar (Eds.), Progress in brain research (Vol. 3; pp. 109–125). Amsterdam, the Netherlands: Elsevier.
Karageorghis, C. I., Bruce, A. C., Pottratz, S. T., Stevens, R. C., Bigliassi, M., & Hamer, M. (2018a). Psychological and psychophysiological effects of recuperative music post-exercise. Medicine & Science in Sports & Exercise, 50, 739–746.
Karageorghis, C. I., Cheek, P., Simpson, S. D., & Bigliassi, M. (2018b). Interactive effects of music tempi and intensities on grip strength and subjective affect. Scandinavian Journal of Medicine & Science in Sports, 28, 1166–1175.
Karageorghis, C. I., Ekkekakis, P., Bird, J. M., & Bigliassi, M. (2017). Music in the exercise and sport domain: Conceptual approaches and underlying mechanisms. In M. Lesaffre, P.-J. Maes, & M. Leman (Eds.), The Routledge companion to embodied music interaction (pp. 284–293). New York, NY: Routledge.
Karageorghis, C. I., Hutchinson, J. C., Bigliassi, M., Watson, M. P. E., Perry, F. A., Burges, L. D., Melville-Griffiths, T., & Gomes-Baho, T. J. G. (2019). Effects of auditory-motor synchronization on 400-m sprint performance: an applied study. International Journal of Sports Science and Coaching, 14, 738–748.
Karageorghis, C. I., & Jones, L. (2014). On the stability and relevance of the exercise heart rate‒music-tempo preference relationship. Psychology of Sport and Exercise, 15, 299–310.
Karageorghis, C. I., Bird, J. M., Hutchinson, J. C., Hamer, M., Delevoye-Turrell, Y. N., Guérin, S. M. R., Mullin, E. M., Mellano, K. T., Parsons-Smith, R. L., Terry, V. R., & Terry, P. C. (2021b). Physical activity and mental well-being under COVID-19 lockdown: a cross-sectional multination study. BMC Public Health, 21, e988.
Karageorghis, C. I., Jones, L., Howard, L. W., Thomas, R. M., Moulashis, P., & Santich, S. J. (2021a). When it HIITs, you feel no pain: Psychological and psychophysiological effects of respite-active music in high-intensity interval training. Journal of Sport & Exercise Psychology, 43, 41–52.
Karageorghis, C. I., Jones, L., Priest, D.-L., Akers, R. I., Clarke, A., Perry, J. M., Reddick, B. T., Bishop, D. T., & Lim, H. B. T. (2011). Revisiting the relationship between exercise heart rate and music tempo preference. Research Quarterly for Exercise and Sport, 82, 274–284.
Karageorghis, C. I., Mouzourides, D. A., Priest, D.-L., Sasso, T. A., Morrish, D. J., & Walley, C. L. (2009). Psychophysical and ergogenic effects of synchronous music during treadmill walking. Journal of Sport & Exercise Psychology, 31, 18–36.
Karageorghis, C. I., & Priest, D.-L. (2012). Music in the exercise domain: A review and synthesis (Part I). International Review of Sport and Exercise Psychology, 5, 44–66.
Karageorghis, C. I., Priest, D.-L., Williams, L. S., Hirani, R. M., Lannon, K. M., & Bates, B. J. (2010). Ergogenic and psychological effects of synchronous music during circuit-type exercise. Psychology of Sport and Exercise, 11, 54–68.
Karageorghis, C. I., & Terry, P. C. (2009). The psychological, psychophysical and ergogenic effects of music in sport: A review and synthesis. In A. J. Bateman & J. R. Bale (Eds.), Sporting sounds: Relationships between sport and music (pp. 15–46). Abingdon, UK: Routledge.
Karageorghis, C. I., Terry, P. C., Lane, A. M., Bishop, D. T., & Priest D.-L. (2012). British Association of Sport and Exercise Sciences expert statement on the use of music in exercise. Journal of Sports Sciences, 30, 953–956.
Kaur, H., Singh, T., Arya, Y. K., & Mittal, S. (2020). Physical fitness and exercise during the COVID-19 pandemic: A qualitative enquiry. Frontiers in Psychology, 11, e293.
Koelsch, S. (2014). Brain correlates of music-evoked emotions. Nature Reviews Neuroscience, 15, 170–180.
Kornysheva, K., von Cramon, D. Y., Jacobsen, T., & Schubotz, R. I. (2010). Tuning-in to the beat: Aesthetic appreciation of musical rhythms correlates with a pre-motor boost. Human Brain Mapping, 31, 48–64.
Leknes, S., & Tracey, I. (2008). A common neurobiology for pain and pleasure. Nature Reviews Neuroscience, 9, 314–320.
Leman, M., Moelants, D., Varewyck, M., Styns, F., van Noorden, L., & Martens, J.-P. (2013). Activating and relaxing music entrains the speed of beat synchronized walking. PLoS ONE, 8, e67932.
Levitin, D. J. (2007). This is your brain on music: The science of a human obsession. London, UK: Atlantic Books.
Lim, H. B., Karageorghis, C. I., Romer, L. M., & Bishop, D. T. (2014). Psychophysiological effects of synchronous versus asynchronous music during cycling. Medicine & Science in Sports & Exercise, 46, 407–413.
MacDougall, H. G., & Moore, S. T. (2005). Marching to the beat of the same drummer: The spontaneous tempo of human locomotion. Journal of Applied Physiology, 99, 1164–1173.
Maes, P.-J., Buhmann, J., & Leman, M. (2016). 3Mo: A model for music-based biofeedback. Frontiers in Neuroscience, 10, e548. doi:10.3389/fnins.2016.00548
Mithen, S. (2005). The singing Neanderthals: The origins of music, language, mind and body. London, UK: Weidenfeld & Nicolson.
Moens, B., Muller, C., van Noorden, L., Franěk, M., Celie, B., Boone, J., Bourgois, J., & Leman, M. (2014). Encouraging spontaneous synchronisation with D-Jogger, an adaptive music player that aligns movement and music. PLoS One, 9, e114234. doi:10.1371/journal.pone.0114234
Nakashima, T., Naganawa, S., Sone, M., Tominaga, M., Hayashi, H., Yamamoto, H., Liu, X., & Nuttall, A. L. (2003). Disorders of cochlear blood flow. Brain Research Reviews, 43, 17–28.
Priest, D.-L., & Karageorghis, C. I. (2008). A qualitative investigation into the characteristics and effects of music accompanying exercise. European Physical Education Review, 14, 347–366.
Ramji, R., Aasa, U., Paulin, J., & Madison, G. (2016). Musical information increases physical performance for synchronous but not asynchronous running. Psychology of Music, 44, 984–995.
Sanchez, X., Moss, S. L., Twist, C., & Karageorghis, C. I. (2014). On the role of lyrics in the music–exercise performance relationship. Psychology of Sport and Exercise, 15, 132–138.
Schneider, S., Askew, C. D., Abel, T., & Strüder, H. K. (2010). Exercise, music, and the brain: is there a central pattern generator? Journal of Sports Sciences, 28, 1337–1343.
Shin, M. (2021). Interactive effect of listening to music and mid- and post-exercise affective change on exercise intention. Psychology, Health & Medicine. Advance online publication. https://doi.org/10.1080/13548506.2021.1960390
Smirmaul, B. P. (2017). Effect of pre-task music on sports or exercise performance. The Journal of Sports Medicine and Physical Fitness, 57, 976–984.
Stork, M. J., Karageorghis, C. I., & Martin Ginis, K. A. (2019). Let’s Go: Psychological, psychophysical, and physiological effects of music during sprint interval exercise. Psychology of Sport and Exercise, 45, e101547.
Stork, M. J., Kwan, M. Y., Gibala, M. J., & Martin Ginis, K. A. (2015). Music enhances performance and perceived enjoyment of sprint interval exercise. Medicine & Science in Sports & Exercise, 47, 1052–1060.
Terry, P. C., & Karageorghis, C. I. (2011). Music in sport and exercise. In T. Morris & P. C. Terry (Eds.), The new sport and exercise psychology companion (pp. 359–380). Morgantown, WV: Fitness Information Technology.
Terry, P. C., Karageorghis, C. I., Curran, M. L., Martin, O. V., & Parsons-Smith, R. L. (2020). Effects of music in exercise and sport: A meta-analytic review. Psychological Bulletin, 146, 91–117.
Terry, P. C., Karageorghis, C. I., Mecozzi Saha, A., & D’Auria, S. (2012). Effects of synchronous music on treadmill running among elite triathletes. Journal of Science and Medicine in Sport, 15, 52–57.
Wilke, J., Mohr, L., Tenforde, A. S., Edouard, P., Fossati, C., González-Gross, M., Sánchez Ramírez, C., Laiño, F., Tan, B., Pillay, J. D., Pigozzi, F., Jimenez-Pavon, D., Novak, B., Jaunig, J., Zhang, M., van Poppel, M., Heidt, C., Willwacher, S., Yuki, G., Lieberman, D. E., Vogt, L., Verhagen, E., Hespanhol, L., & Hollander, K. (2021). A pandemic within the pandemic? Physical activity levels substantially decreased in countries affected by COVID-19. International Journal of Environmental Research and Public Health, 18, e2235.
Zatorre, R. J., & Salimpoor, V. N. (2013). From perception to pleasure: Music and its neural substrates. Proceedings of the National Academy of Sciences, 110, 10430–10437.